As the installed costs for solar PV projects have rapidly declined in recent years, more solar PV has been installed across the U.S., and the traditional model of a centralized utility grid is evolving to accommodate distributed energy resources (DER). These changes are empowering utility and large commercial customers to strategically manage energy and control costs by tapping systems that can make renewable power available when the sun is not shining and energy demand is high. Microgrids, which strategically manage DER, can make new revenue streams available and allow users to sustain power even when the grid is off due to a power outage or weather-related event.
What is a microgrid?
Microgrids are standalone electrical power systems that consist of multiple generating assets and often storage sources supplying loads that can be powered independent of the primary utility transmission and distribution grid. The three basic operating modes that are typical of microgrids include the following:
- Normal grid connected operation: During normal operation, the microgrid is connected to the grid and the loads are powered by a mix of grid and DER power. Local DER assets may be running behind the meter on the site.
- Islanded operation: During islanded operation, the microgrid system is not connected to the grid and the load is powered by the DER independently, off the grid.
- Outage mode (or black-start mode): When generation type and size are not adequate for seamless islanding, normal connection to the grid is suspended, resulting in a blackout and island mode operation being started with proper sequencing of generators and loads. This could occur for a variety of reasons. For example, due to DER size or lack of load shedding, the DER may not be able to handle the load.
In most current designs, the microgrid is tied to the upstream grid via a point of interconnection (POC) and is managed by various controller architectures, which are the “brains” of the operation. This local control of assets enables faster, semi-autonomous or autonomous control of the microgrid devices to better maintain operation within connected equipment limits.
Beyond the controller architecture and POC, microgrids include a physical connection between the distributed energy resources and loads. These system components typically include grid connection medium-voltage switchgear, medium-voltage transformers, low-voltage switchgear or switchboards, local AC and DC switching and protection, metering and the control interface with the microgrid controller.
Determining if a microgrid makes sense
Microgrids can provide power resiliency and financial payback. Yet, how do you know if it is the right solution for a specific application? And what is the right size and design for your application? Microgrids are not a one-size solution that fits all circumstances. Many factors need to be explored, among them the existing electrical infrastructure (if any), load profile and growth, utility rates, existing generation assets, generator control capabilities, etc.
A feasibility study is used to identify and define the microgrid project for optimal technical features (use cases) and economic return. Qualified vendors have evolved routine methods to assess the full breadth and depth of standard microgrid concerns while defining the unique and specific details appropriate for each potential implementation case.
A feasibility study should attempt to answer in very simple terms whether a microgrid makes good sense to employ in a specific circumstance and, if so, what configuration and components are optimal to meet the specific power needs of the given scenario. The feasibility study process walks customers through these concerns step-by-step, first determining critical needs and requirements, then developing a microgrid plan and finally outlining more specific technical aspects and recommendations.
Initial screening questions might touch upon functionality requirements; existing load and generation information; automation infrastructure availability; utility requirements; generation preferences; and other security, legal and commercial aspects.
For example, the screening might attempt to uncover functional specifications by exploring critical load requirements as well as any load shedding, demand response or black-start (outage mode operation) needs.
Peak electrical and thermal load profiles, load types and profiles must also be identified prior to the design. During the screening, further assessment of existing generation and automation systems, including their scope, functionality and interfaces, should be pursued in-depth to determine more specific microgrid assets and system topology.
Each critical asset (facilities and present applications) must be identified and addressed in the study in terms of its needs and the criticality of each asset. Based on these identified power-critical assets, load sizes and profiles, the location of supply and storage infrastructure necessary to adequately support critical assets will be identified and designed into the proposed system.
The feasibility study is more than an exercise in determining the technical aspects. It should also cover an economic analysis so that it is clear if a microgrid solution is economically feasible. Additionally, it should support documentation of utility requirements inclusive of billing, interconnection and application requirements, SCADA communications, ownership agreements, metering contracts, permits, incentives and other environmental concerns.
With all analysis complete, a comprehensive and tailored energy reliability plan can be developed for the microgrid implementation. The resulting feasibility report should comprehensively detail the intended functionality of the proposed microgrid and its scope; existing system assets to serve the load profile; a proposed design; details on DER, including renewable energy sources; recommendations for suitable energy storage technologies and their sizing based on the microgrid requirements; an overview of operational modes and control strategies within the design; and detailed cost estimates for ascertaining benefits.
Simulating microgrid operation to better understand benefits
How can you be sure the microgrid will perform as intended before you cut the ribbon on the project? Even if performance and functionality can be verified in advance, what if you could go a step further and analyze the data needed to improve performance before the system is installed?
Developing a microgrid requires power system, automation and control knowledge well beyond the specific energy resources and equipment involved. Seeing the big picture and understanding how to optimize the overall system is a critical aspect to microgrid success.
Feasibility studies can do more than provide the sizing of equipment and assess the functionality and likely future performance characteristics. The last compelling aspect of a feasibility study is the ability to see how the system functions before ground is broken on the project. This microgrid simulation can provide insights on the feasibility, design and application in a virtual environment.
The simulations of complex microgrid systems can enable owners and project designers to understand the system as if the assets and devices were already connected. This demonstration informs how the system is configured and optimized during the design phase. The ability to play with the microgrid system before it is in place helps drive a better understanding of the system dynamics and feasibility – all based on data from the simulation. Simulations also give owners confidence that the system will perform as expected, as well as data-driven insights to modify the design to optimize performance.
A Sample Microgrid Simulation Result
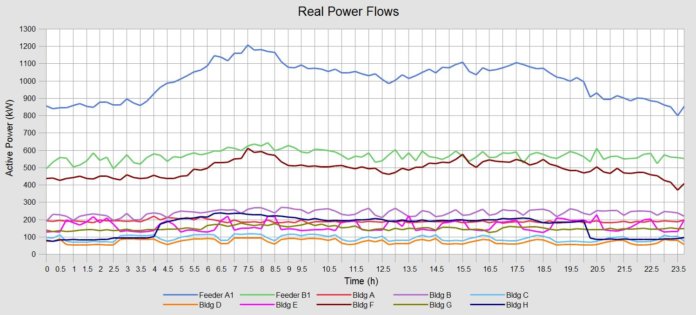
Accurate simulation results lead to more precise planning and management of the microgrid system, including its distributed generation and distributed energy resources. This allows projects to move more quickly and successfully, with confidence they are optimized out of the gate.
Microgrids to advance solar and other DER
Renewable energy is intermittent by nature. The sun does not shine on demand, and to maximize renewable energy generation, we need to make the most of what we get. As renewable energy penetration continues to expand, finding the best ways to store and distribute energy is increasingly important to accommodate those cloudy, rainy days. This is true for both the utilities that deliver electricity and the organizations investing in solar PV.
As more and more renewables come online, energy developers and utilities need to consider various forms of stored energy to fully harvest the potential of renewable resources. Additionally, microgrids help to maintain line parameters that may otherwise be compromised without energy damping. Energy harvest from large-scale PV systems has variations that do not match up with utility loads. Intelligent microgrid systems with energy storage devices can help match up generation to load while maximizing energy production and help maintain frequency and voltage tolerances.
Microgrids and energy storage are helping combine solar and other DER to advance power resiliency and open new revenue streams and reduce energy costs. In effect, microgrids can further accelerate the adoption of distributed renewable energy sources that are intermittent – reducing global dependence on fossil fuels while lowering climate-damaging carbon emissions.
However, feasibility studies, system analysis and simulation are critical first steps to determining requirements such as the addition of new DER and storage assets, load profile and growth, utility rates, existing on-site generation, and generator control capabilities.
John Vernacchia is the segment manager for renewable energy solutions within Eaton’s electrical business. Vernacchia is a member of the IEEE Industry Applications and Power & Energy Societies and holds a bachelor of science in mechanical engineering from Virginia Tech.
Main photo: Eaton’s virtual microgrid managed by its Power Xpert Energy Optimizer controller. The system pictured is installed at the company’s Power Systems Experience Center in Pittsburgh.